What Is Total Internal Reflection Fluorescence (TIRF) Microscopy & Is It Right For You?
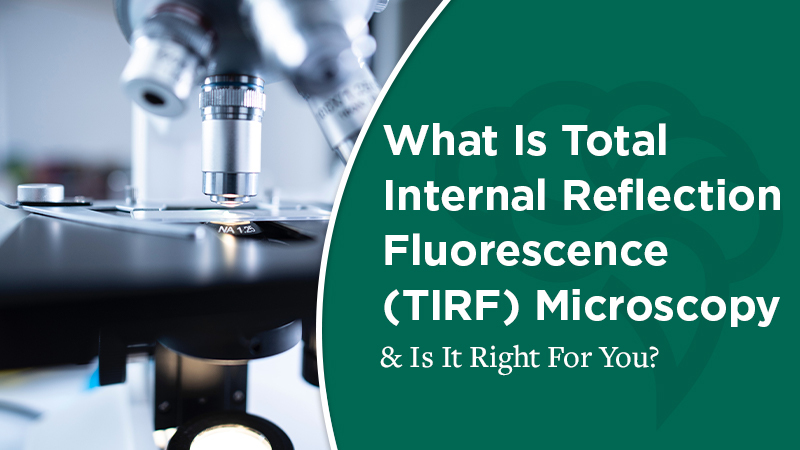
TIRF is not as common as other microscopy based techniques due to certain restrictions. We will discuss these restrictions, then analyze why it might be perfect for your experiment.
TIRF relies on an evanescent wave, created through a critical angle of coherent light (i.e. laser) that reaches a refractive index mismatch.
What does it mean in practice?
A high angle laser reflects off the interface of the coverslip and the sample. Although the depth that this wave penetrates is dependent on the wavelength of the light, in practice it is approximately 50-300nm from the coverslip. Therefore, the cell membrane is often the focus of TIRF microscopy.
Yet another restriction is the ability to do multichannel images. Since the depth of imaging is dependent on the wavelength of light; the angle of illumination needs to be different for each wavelength of light used. Modern commercial systems can make those adjustments, but if the system is not well calibrated it could create artifacts of colocalization in the z-direction.
Figure 1: Illumination of a sample by widefield, laser scanning confocal, and TIRF. Widefield microscopy illuminates the entire sample (red), while laser scanning confocal microscopy (LSM) directs light to a pinpoint and illuminates cones of light through the sample. TIRF microscopy only illuminates the thin section being imaged.
TIRF Provides An Increase In Signal-To-Noise Ratio (S/N)
Out of focus light creates a haze in an image, which diminishes the ability of a system to resolve two points of light or structures. In widefield microscopes the whole sample is illuminated and then a section of the sample is collected. All the out of focus light is also collected during the acquisition (Figure 1). In confocal microscopy, a pinhole is used to focus the light into a cone and that light is collected from the focal plane and excluded from elsewhere. TIRF is unique as only the area of acquisition is illuminated and collected. Compared to widefield microscopy, TIRF can provide over a 30x increase in S/N.
In an ideal system, the resolution is approximately half of the wavelength of light. Unfortunately, we don’t live in the perfect physics scenario that is needed for the calculation. Out of focus light increases the distance between two points needed to resolve the structures. Since TIRF reduces out of focus light, it increases the effective resolution of the system.
Figure 2: The effect of S/N on resolution of an image. Resolution is the ability to distinguish between two points of light. Low S/N obscures details (left), while a high S/N provides an image with clear structures in the sample (right). Leica Microsystems
TIRF Is Gentle On Live Samples
The evanescent wave created for imaging is at most 300nm deep, allowing most of the cells to remain in the dark. This is important because when cells have fluorophores present, the light causes the creation of ROS, specifically singlet oxygen 1O2 which causes cell damage and altered physiology. This phenomena is called phototoxicity and ranges from cell death to phenotype artifacts. Therefore, it is particularly critical to limit phototoxicity of cells if you are imaging samples over an extended period of time.
TIRF Minimizes Photobleaching
The limited depth of illumination and acquisition in TIRF work to our advantage when it comes to photobleaching. The unilluminated area of the cell can provide new fluorescent units over time, decreasing photobleaching. For example, actin can either be monomeric or filamentous, it readily transitions between the two forms. If you are imaging actin formation at the interface of the cell membrane, new actin monomers will be constantly brought in, providing fresh fluorescent proteins.
TIRF Acquisition Time Is Faster Than Confocal Microscopy
TIRF acquisition occurs at the same rate as widefield microscopy, so it can capture very rapid time series. Laser scanning confocal microscopy (LSM) is much slower, and even spinning disc confocal microscopes are somewhat slower than TIRF. Since several cellular signaling events occur at the millisecond level or faster, therefore speed is critical for these experiments.
Best Applications For TIRF Microscopy
TIRF is a very popular technique for experiments involving cellular processes at the cell membrane. One such experiment is investigating G-protein coupled receptor (GPCR) signaling, which is an important family of signalling receptor proteins for cells to sense the extracellular environment.
Another popular use for TIRF microscopy is imaging endocytosis/exocytosis. Under widefield microscopy conditions, there are too many fluorophores at different z-positions so scientists can obtain the resolution needed to observe single events. TIRF on the other hand takes very thin sections allowing the puncta to be observed. LSM can provide thin sections, but is too harsh and slow for experiments such as neurotransmitter release in synapse.
Figure 3: Cell Focal Adhesions imaged by (a) widefield (b) TIRF. Notice how the haze has been removed allowing for the resolution of the focal adhesions. Nikon MicroscopyU
The last category of experiments that are popular with TIRF microscopy is cytoskeletal dynamics (actin, tubulin, and intermediate filaments). TIRF microscopy is an excellent technique for focal adhesions, cellular locomotion, and protein trafficking along the microtubules. The cytoskeleton often has many overlapping filaments in the z-direction, so the thin imaging section really increases the ability to resolve single filaments. Figure 3 demonstrates the improvement in S/N in focal adhesions.
Concluding Remarks
TIRF provides a 30x increase in the S/N ratio facilitating a much higher resolution. It also has the advantage of being gentle on the live samples and does not spark any altered physiology due to ROS buildup. Reduced photobleaching effect and faster acquisition time favor TIRF use for studying cell signaling events. TIRF microscopy certainly has its limits due to the method of illumination, but the same illumination makes it adept for numerous cell biology experiments.
To learn more about important techniques for your flow microscopy lab, and to get access to all of our advanced materials including 20 training videos, presentations, workbooks, and private group membership, get on the Expert Microscopy wait list.
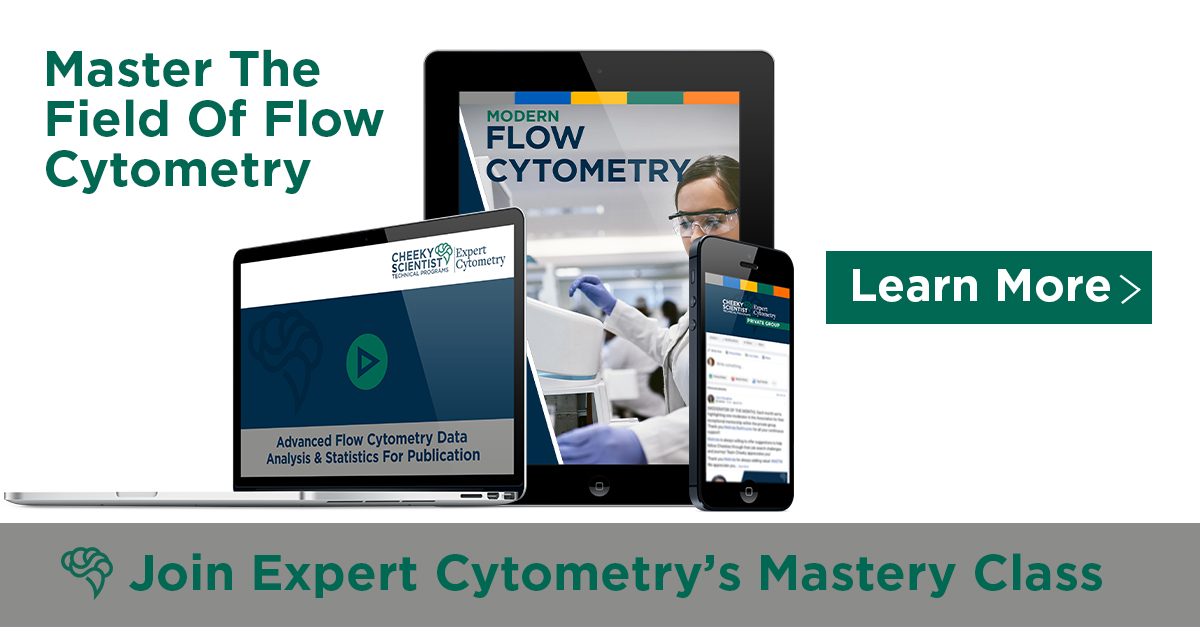
ABOUT HEATHER BROWN-HARDING
Heather Brown-Harding, PhD, is currently the assistant director of Wake Forest Microscopy and graduate teaching faculty.She also maintains a small research group that works on imaging of host-pathogen interactions. Heather is passionate about making science accessible to everyone.High-quality research shouldn’t be exclusive to elite institutions or made incomprehensible by unnecessary jargon. She created the modules for Excite Microscopy with this mission.
In her free time, she enjoys playing with her cat & dog, trying out new craft ciders and painting.You can find her on twitter (@microscopyEd) a few times of day discussing new imaging techniques with peers.
More Written by Heather Brown-Harding