7 Individual Artifacts In Fluorescence Microscopy And How To Minimize Them
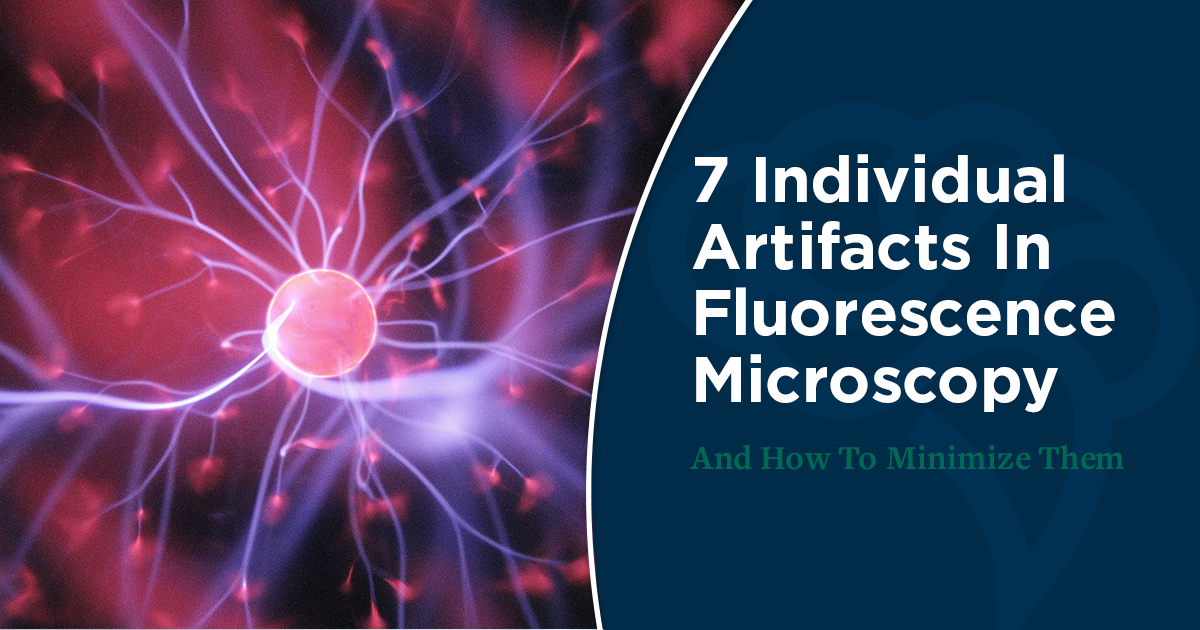
There are 7 different “artifacts” that may be affecting the quality of your imaging. Before digging into the details, let’s begin by defining an artifact: Essentially, it is any error introduced through sample preparation, the equipment or post-processing. This is an important concept to grasp because the effect of artifacts can cause false positives or negatives, and they can physically distort your data. This is, of course, at odds with your mission to obtain reliable quantitative data.
So what can you do to stop these artifacts? The problems can range from dirty objectives to bigger issues like light path aberrations. We’re going to discuss 7 individual artifacts and how to minimize them.
1. Sample preparation.
Sample preparation presents a few different opportunities for artifacts to arise. Air bubbles are a problem because they cause a difference in brightness through light distortion. This occurs due to the differences in refractive index bending light differently through the sample and back to the objective.
Another common sample-prep problem is crushing. If you’re not very light with your coverslips, you can actually compress a 3D structure and cause breakage within the sample – breakage that’s not actually there if it was still a live organism.
Overall, caution is heavily recommended during your sample preparation process. You must ensure that your fixation is full and that you have as few air bubbles as possible. Although if you do have a few bubbles in a location apart from the precise area you need to image, simply image elsewhere. As for crushing, add a little gasket around your structure to ensure it doesn’t get crushed and make sure not to move the coverslip after it has been placed.
For more information about critical sample preparation see our blogs on coverslips and controls.
2. Lightpath aberrations.
Lightpath aberrations are those that actually occur within the microscope system you are using to image your sample. There are 3 main causes of aberrations that different types of objectives can correct. https://www.microscopyu.com/microscopy-basics/introduction-to-microscope-objectives1. Chromatic aberration.
Chromatic aberration is caused by a spherical lens bringing different wavelengths of light into focus at different levels. You can think of a prism spectrally filtering out white light into multiple colors. Thus, you get multiple images superimposed on top of each other. When you have chromatic aberration, it can be corrected with apochromatic objectives. 2. Spherical aberration.
In the case of a spherical aberration, the optical quality of the image is lessened because the center of the lens has slightly different qualities than the edges. This causes the rays of light to not converge on one point. All modern objectives from the major microscope brands will have some sort of correction and are referred to as an aspherical lens. As with its chromatic cousin, this defect can be corrected using an apochromatic objective for multicolor fluorescent imaging. 3. The curvature of the field.
Field curvature causes the image to be centrally focused, but you get out of focus peripherally – or vice versa. A lot of times, this looks like a fisheye lens. A curvature defect is corrected using planar objectives, so look for an objective that starts with “Plan” at the beginning of the name.
Certain other aberrations can occur due to front lens damage and are more difficult to correct. This usually happens when you aren’t correctly taking care of your objectives. Crashing an objective into a stage causes scratches as well as changes in the alignment of the lenses within the objective. Sample slides and fluorescent dots are the best way to check for these problems. The effects can include:
- Stigmatism, in which case everything is just slightly out of focus.
- Comatic aberrations that are recognizable by its resemblance to little comets (if you have very small points of light).
- Geometric distortions, which can stretch your sample in weird directions https://en.wikipedia.org/wiki/Distortion_(optics)
3. Vibration.
Vibration may result from something like a local subway/train, a nearby centrifuge with imperfect alignment, or even just people walking by. These may seem like small vibrations to us, but for a microscope that is trying to produce an image with micrometer pixels, these are large movements. Regardless of the source, vibration leaves you with shaky, unclear, and ultimately unusable images. Users with sensitive systems often combat this using an air table, which actually floats the tabletop on pressurized air and isolates it from nearby vibrations!
Unfortunately, the air table method is not foolproof. Be sure that you place no more than is necessary on your air table. Objects like heaters, for instance, are notorious for causing vibrations due to their blowing fans. It may seem like certain vibrations are too tiny to have any real impact, but when we’re talking about imaging at the microscale, these minuscule vibrations can affect your image quality significantly.
Image of nuclei on a laser scanning confocal microscope, when vibrations were present. Notice the regularly spaced choppy lines.
4. Signal-to-noise deterioration from ambient light.
“Ambient light” refers to things like room light, which will add unspecific illumination that can be captured by your camera or detector. Since the light doesn’t come from a single point, it shows up as background noise in your imaging. This most commonly happens when people are using the fluorescence on a microscope and leave the lab light on, leading to more background and maybe even the occurrence of undesired features.
The simplest solution is to turn off the room lights! But if you can’t do that (maybe you have large windows or you’re in a shared space), you can use black fabric to create a tent around the microscope. This simple step can greatly improve your image by lowering noise to increase your signal-to-noise ratio.
5. Photobleaching.
Photobleaching is the photochemical destruction of a fluorophore, which results in the intensity of your signal decreasing over time. This is important when you’re using fluorescence intensity as a quantitative readout of your experiment. Thus, when planning your experiment you want to look for the most photostable dyes and proteins possible. When you are performing your experiment use the lowest illumination power you can or shorter exposures to minimize photobleaching.
If your experiment can’t prevent photobleaching in a live-cell imaging experiment, you can still create a photobleach curve. This photobleach curve can be applied to your experiment to correct for any loss in intensity. Finally, if you’re doing fixed-cell imaging, you can use mounting media with an anti-fade added to it, which will help combat the photobleaching.
6. Phototoxicity.
Phototoxicity is the damaging of macromolecules due to light illumination. This mainly occurs via the creation of reactive oxygen species (AKA free radicals) that damage DNA and/or proteins. Not unlike the case with photobleaching, you can minimize this artifact by using lower illumination and shortening the exposure.
Another option is that you can also use fluorophores with longer wavelengths. Longer wavelengths of light have less energy, and are therefore less likely to induce ROS formation. Phototoxicity is only a problem for live-cell imaging experiments. More information on how to properly set up a live-cell imaging experiment was covered here.
7. Bleed-Through.
Example of microtubules (green) appearing in DAPI channel (cyan)
Bleed-through, also known as spectral crosstalk, is the excitation of more than 1 fluorophore at a time. This is a problem for colocalization studies or any quantitative measurements, but it also affects overall image quality. To minimize bleed-through, use probes as far apart on the spectrum as possible.
Additionally, during acquisition, use a smaller emission window or cubes with a smaller bandpass. These measures ensure that you’re only exciting and detecting that which you desire. For example, something like a wide-spectrum FITC/TRITC cube may have problems with red and green bleeding into each other. This is because fluorophores don’t have just a single excitation and emission – they can be excited with other cubes or lasers.
Spectral emission of GFP. Due to the wide emission curve, the TRITC filter can also capture GFP emitted light. If GFP is more intense than your RFP, this will appear as “bleed-through”
There are a variety of artifacts that can occur when using a microscope. Some artifacts can be reduced by careful planning of your experiment and proper controls. Artifacts from the system need to also be addressed.
Lenses can be corrected for visual aberrations, and the optical system can be aligned. This can be as a simple is correcting for Kohler illumination, or it can be a little more difficult, like having your lasers aligned. However, proper care of objectives is generally the best preventative tool in your arsenal. In any case, one ought to regularly undertake preventative maintenance on any microscopes used. Artifacts are significant threats to the reliability of your data, but by using the prescribed methods and tools discussed above, you can keep them at an absolute minimum.
Further reading:
To learn more about 7 Individual Artifacts In Fluorescence Microscopy And How To Minimize Them, and to get access to all of our advanced microscopy materials including training videos, presentations, workbooks, and private group membership, get on the
Expert Microscopy wait list.
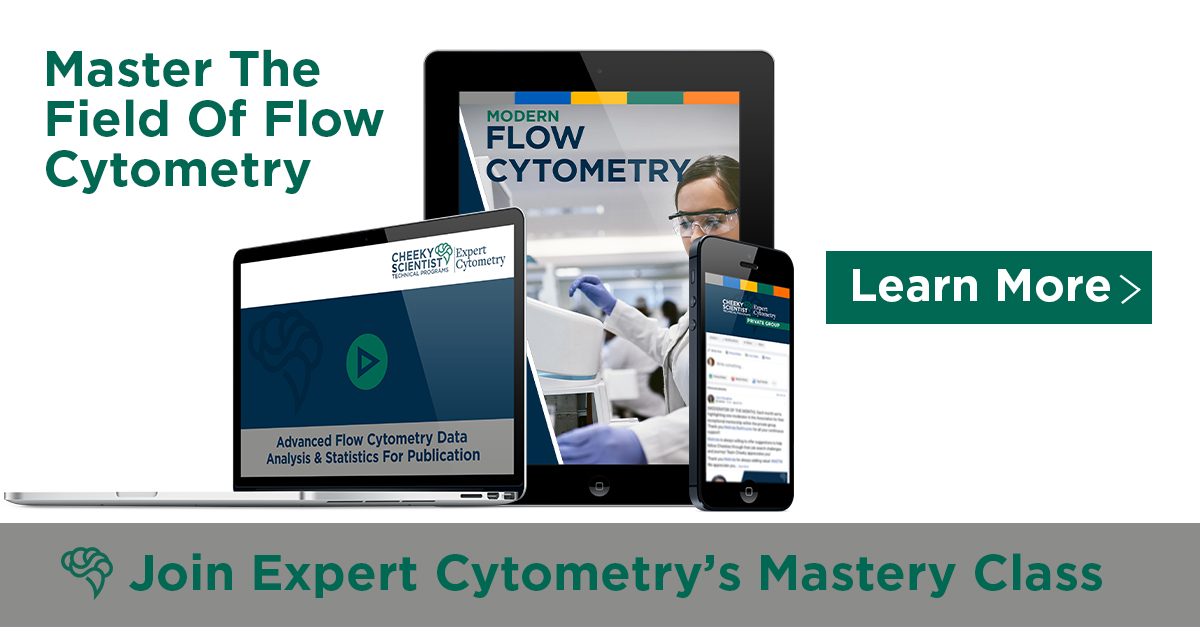
ABOUT HEATHER BROWN-HARDING
Heather Brown-Harding, PhD, is currently the assistant director of Wake Forest Microscopy and graduate teaching faculty.She also maintains a small research group that works on imaging of host-pathogen interactions. Heather is passionate about making science accessible to everyone.High-quality research shouldn’t be exclusive to elite institutions or made incomprehensible by unnecessary jargon. She created the modules for Excite Microscopy with this mission.
In her free time, she enjoys playing with her cat & dog, trying out new craft ciders and painting.You can find her on twitter (@microscopyEd) a few times of day discussing new imaging techniques with peers.
More Written by Heather Brown-Harding